Oleg Astafiev
(National Physical Laboratory, Teddington, UK , Department of Physics, Royal Holloway University of London, Egham, UK, Moscow Institute of Physics and Technology, Dolgoprudny, Russia, Skolkovo Institute of Science and Technology, Moscow, Russia)
The Coherent Quantum Phase Slip (CQPS) effect in superconducting nanowires is exactly dual to the Josephson effect – tunneling of Cooper pairs through a thin dielectric layer. CQPS has been experimentally proven for the first time in Ref. 1 and since then reproduced in different materials [2, 3]. CQPS is interesting from the fundamental point of view as well as for practical applications, particularly, for quantum metrology as it promises to build quantum current standards dual to voltage standards on the Josephson effect. An important milestone in this direction is the demonstration of interference of a pair of flux tunneling amplitudes similarly to charge tunneling amplitudes in SQUIDs.
We demonstrate a device exactly dual to SQUID, which we call a charge quantum interference device (CQUID) [4]. The two nominally identical nanowires with constrictions separated by a superconducting island are fabricated to observe the quantum interference of two flux tunneling amplitudes. The effective tunneling energy depends on the gate induced charge on the island. In our experiment, the CQUID is incorporated into a superconducting loop, forming a phase-slip qubit with tunable energy. Such a circuit allows to measure the CQPS energy using spectroscopic methods. This is exactly dual to the charge qubit with a SQUID, where the effective Josephson energy is tuned by an external magnetic flux.
Figure 1 demonstrates observed oscillations of energies of CQPS qubit with two junctions (nanowires with constrictions) as a function of gate induced charge. The oscillations are not harmonic and well described by the interference effect of two CQPS amplitudes.
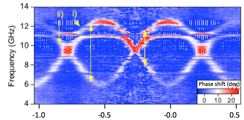
1 O. V. Astafiev, et. al. Nature 484, 355 (2012).
[2] J. T. Peltonen, et. al. Phys. Rev. B 88, 220506 (2013).
[3] J. T. Peltonen, et. al. Phys. Rev. B 94, 180508(R) (2016).
[4] S. E. de Graaf, et. al. Nature Physics 14, 590 (2018).
S. E. de Graaf
(National Physical Laboratory, Teddington, UK)
S. T. Skacel
(Physikalisches Institut, Karlsruhe Institute of Technology, Karlsruhe, Germany)
T. Hoenigl-Decrinis
(National Physical Laboratory, Teddington, UK , Department of Physics, Royal Holloway University of London, Egham, UK)
R. Shaikhaidarov
(Department of Physics, Royal Holloway University of London, Egham, UK , Moscow Institute of Physics and Technology, Dolgoprudny, Russia )
H. Rotzinger
(Physikalisches Institut, Karlsruhe Institute of Technology, Karlsruhe, Germany.)
S. Linzen
(Leibniz Institute of Photonic Technology, Jena, Germany)
M. Ziegler
(Leibniz Institute of Photonic Technology, Jena, Germany)
U. Hubner
(Leibniz Institute of Photonic Technology, Jena, Germany)
H.-G. Meyer
(Leibniz Institute of Photonic Technology, Jena, Germany)
V. Antonov
(Department of Physics, Royal Holloway University of London, Egham, UK, Skolkovo Institute of Science and Technology, Moscow, Russia)
E Il’ichev
(Leibniz Institute of Photonic Technology, Jena, Germany, Russian Quantum Center, National University of Science and Technology MISIS, Russia)
A. V. Ustinov
(Physikalisches Institut, Karlsruhe Institute of Technology, Karlsruhe, Germany, Russian Quantum Center, National University of Science and Technology MISIS, Russia)
A. Ya. Tzalenchuk
(National Physical Laboratory, Teddington, UK, Department of Physics, Royal Holloway University of London, Egham, UK)
Oleg Astafiev
(National Physical Laboratory, Teddington, UK , Department of Physics, Royal Holloway University of London, Egham, UK, Moscow Institute of Physics and Technology, Dolgoprudny, Russia, Skolkovo Institute of Science and Technology, Moscow, Russia)
There are no materials yet.